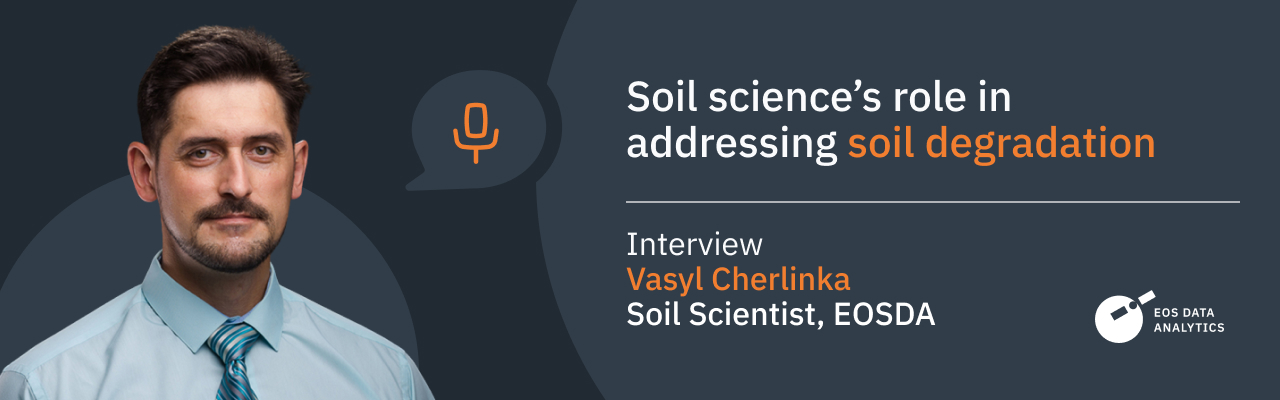
The Role Of Soil Science In Mitigating Soil Degradation
Soil is the foundation of all food production processes, with nearly 95% of global food production dependent on it.
In the interview, Vasyl Cherlinka, Soil Scientist at EOS Data Analytics, discusses the problem of rational soil use, soil degradation, its causes and consequences for global food security. The specialist also talks about digital soil mapping and modeling and their role in monitoring and sustaining soil health and maximizing crop yields.
What Is Soil Degradation?
Soil degradation is the loss of its physical, physiochemical, or biological characteristics due to natural or anthropogenic processes. There are four main reasons for soil degradation.
The first cause is the pathology of the soil profile and genetic horizons. Genetic horizons are soil layers with certain features (e.g., texture, color, structure, thickness, hosted biological communities, chemical and mineral content). Each layer’s properties indicate it has been exposed to given soil-forming processes. And a soil profile is how these horizons are arranged.
Soil erosion — detachment, movement, and deposition of topsoil due to wind or water — is one of the cause types. Although the reasons for soil erosion are natural, people can exacerbate them, for example, during farming activities like plowing or arranging and cultivating crops along slopes.
Soil compaction happens when its particles are pressed together, and pore space between them reduces. As a result, less water and air penetrate the soil, which is bad for plant and root system development. Massive tractors (especially wheeled ones) that can press soil up to one and a half meters can lead to soil compaction.
Pollution and chemical poisoning also threaten soil health. First, I’m talking about the uncontrolled use of mineral fertilizers that can change the soil pH. The second issue is that an excessive amount of fertilizer not only drives crop growth but also makes crops toxic for humans. Everyone probably heard about nitrates: eating nitrate-rich food may lead to heart muscle paralysis.
One should be careful with pesticides. These chemicals, popular among agrarians, can be used for weed control (herbicides), prevention of fungal plant diseases (fungicides), and fighting pests (zoocides, insecticides, etc.). When applied correctly, they save more than 30% of the crop. But surplus pesticides can migrate and enter groundwater. By accumulating in the soil, they can be transmitted through food chains and cause disease to animals and people.
Finally, big fertilizer doses can get to nearby bodies of water with an ongoing water flow, and excessive concentration of inputs in water leads to such a phenomenon as eutrophication of water basins. That’s what happens to the Dnipro river in Ukraine in summer: its waters turn green due to growing algae that use lots of oxygen from the water, and the fish die. So, improper use of these inputs causes a chain of adverse events.
The third reason for soil degradation is the violation of its water and chemical regimes. It can be indicated as desertification, landslides and mudslides, secondary salinization etc.
Several environmental changes lead to desertification. Climate change causes decreasing rainfall. Climate zone boundaries are shifting (changes in fauna and flora also show this shift), hence, fertile soil loses its features, and territory turns into desert.
Landslides and mudslides, typical for mountainous and hilly areas, can be triggered by heavy rainfalls, volcano eruptions, earthquakes, road pavings, mining, or deforestation.
The anthropogenic destruction of forests (one tree can hold up to 500 liters of water during the rain) and shrub vegetation lead to profound changes in the water regime of territories. Under these conditions, soil masses begin to shift over wetted clays, sliding down the slope, and the soil cover is completely destroyed.
The soils of exposed slopes (without vegetation) are washed away so intensively that all genetic horizons disappear in 3–5 years, and only rocks lie at the surface. The water stream, which first washed out a significant part of fine material from the surface of sloping soils, having gained speed, sweeps away everything in the mountain valleys in its path: bridges, fields, gardens, herds of animals, settlements. This event was called a mudflow.
There may be secondary salinization (the release of salts from lower soil layers to the surface due to improper irrigation regime), change in nature and the appearance of secondary acidity (change of balance in acidity that can be caused by the wrong choice of crops or fertilizers), and soil dehydration.
The fourth cause is the violation of the bioenergy regime of soils and ecosystems, and it can be expressed in devegetation and dehumification (decomposition of organic matter). The former means vegetation decline, and it can occur because of the disrupted soil water balance in a given territory. Devegetation can be driven by wind blowing off the soil where the remaining plants grow. Soils with artificially depleted vegetation lose their root biomass. Therefore their reserves of valuable minerals, organic matter, and bioenergy resources become depleted. Such soils turn sterile, structurally unsound, and can easily erode. It happens in Ukraine’s southern part, for instance.
Dehumification is the loss of soil organic matter amid a long-term non-use of mineral and organic fertilizers, the influence of erosion processes, and other factors. Organic substances making up organic matter begin to decompose under the influence of the soil’s microbial part. Soil organic matter stabilizes soil acting like a glue keeping its particles together. The smaller the percentage of humus in the soil, the greater the risk of soil degradation due to natural or anthropogenic reasons.
Intensive agriculture practices are also to blame for soil degradation. For instance, those who grow the same plants season after season without a break gradually deplete the soil. This phenomenon has been known for a long time and is called soil fatigue. Fertilizers won’t help to restore soil fertility in this case.
Experts Think Soil Degradation Threatens Global Food Security. Do You Agree With This Notion?
I have to agree with them because the world population is growing, and the amount of natural resources is not: land and soil are limited due to territorial features of the planet Earth. About 71% of the planet’s surface is covered with water, with oceans holding nearly 96.5% of all water. Mountain systems make about 40% the land surface. A significant area is located in polar regions and other areas unsuitable for agriculture (deserts, semi-deserts, landfills, etc.). Over the years, less and less lands remain available for farming. Large territories are withdrawn from agricultural circulation amid urbanization, and growing desertification worsens things. Given that over 95% of food production depends on the soil, people must preserve it to be able to feed themselves in the future.
What’s alarming is ineffective and unsustainable soil use. The problem is severe in African countries and Latin America. For instance, soil on arable lands in Brazil is exposed to water erosion as nearly 2000 millimeters of precipitation fall in the county yearly. There is also systematic deforestation that adds pressure on it.
But it’s not only soil degradation that puts food security at risk (I know, it’s off-topic.) Military conflicts are also a threat, resulting in disrupted agricultural supply chains. In 2022, the world sees how the Russian invasion of Ukraine is causing a food shortage. Currently, two of Ukraine’s ports used for grain exports, Mariupol and Kherson, are blocked by Russia. Ukraine’s role in supporting certain countries’ food security is hard to underestimate: in 2021 alone, the country exported 10% of wheat. The top three buyers were China, Egypt, and Turkey.
It’s difficult even to assess the damage caused to the soil by war. Suffice it to list the main negative effects that complement the previously remembered degradation.
Soil is physically damaged due to:
- violation or removal of soil material due to counter-offensive attempts
- missiles, bombs, shells, installation of anti-tank and anti-personnel mines
- combat traffic, including maneuvers of heavy wheeled and tracked vehicles
- provoked fires in agricultural or forestry lands
There is also a chemical effect of military action on soils, such as contamination with potentially toxic elements, pollution by energy compounds, and the influence of combat chemicals.
Join the EOSDA Partner Program
So, To Preserve Soils, Farmers And Agricultural Holdings Must Sustain Soil Health By Using Optimum Amounts Of Fertilizers, Managing Irrigation, Or Rotating Crops. Governmental Agencies Must Control How Individuals And Businesses Use Natural Resources. What’s The Role Of Scientists?
Agricultural production includes two main sectors — crop production and animal husbandry, which have different shares depending on the country. But both industries directly affect the soil. The crop production’s task is the cultivation of agricultural plants that convert the solar radiation energy into the energy of organic matter, that is, different types of crops. The cornerstone for the successful development of animal husbandry is a solid forage base, the creation of which is impossible without crop production. Therefore, the relationship between crop and livestock industries is the basis for rational organization and effective agricultural production management.
Since soil is the basis of all production processes, scientists have been focusing on finding methods to maximize its beneficial properties (increase fertility) and minimize harm (prevent degradation of various soil kinds). Therefore, the soil is the primary means of production since it is both an object and a labor means.
Thanks to researchers’ efforts, agriculture as a science appeared. Agriculture allows for producing food for people, feed for the livestock industry, and raw materials for the food processing sector.
Agriculture encompasses numerous disciplines like plant growing, vegetable growing, fruit growing, viticulture, selection, grassland growing, etc.
It should be noted that soil science isn’t included in the mentioned list of sciences. However, agriculture benefits from its achievements.
As you can see, there is a close interaction between a whole range of sciences to achieve the maximum effect from soils regarding crop yields. Even at the beginning of the last century, a wheat yield of 15–20 centners per hectare was considered excellent. Today, 50–60 centners per hectare is a mediocre result, and the best farms receive 100 and even 120. And such massive growth is observed in almost all crops. The merit of scientists here is invaluable!
There are several methods for improving soil fertility: chemical (e.g., fertilization researched within agrochemistry), physical and biological. And agriculture focuses on the last two of them. The rational use of arable land and soil fertility improvement involve a complex of biological, chemical, and physical methods of influencing the soil.
All these activities affect the environment, the quality of products, human health, and all life on earth. Therefore, it’s crucial to control their correctness and study possible consequences. That’s what scientists are doing.
Besides introducing control and monitoring systems, the solution to this problem is facilitated by improving zonal farming systems and introducing soil-protective technologies for growing plants based on modern methodological principles of soil fertility management.
Our modern, to a large extent, is like this thanks to the efforts of scientists who study and develop methods for regulating the soil’s water-air, thermal, and nutrient regimes. They aim to define optimal plant growth and development conditions, create weed control measures, rational crop rotations, and tillage systems. They also research methods of rational land use, its protection from erosion, and obtaining high and sustainable yields with a constant increase in soil fertility.
Please Tell Us About Digital Soil Mapping
Modeling means analyzing ground, satellite, or air data using a wide range of algorithms or software (GIS, R, Python, etc.) that has these algorithms under the hood to create a map of soil types or characteristics. At the same time, depending on the task, you can create static (current, past, or future state) or dynamic maps.
What Are The Benefits Of Digital Soil Maps?
The advantage of digital soil maps lies in their unrivaled accuracy. Maps modeled based on a mathematical approach are far more accurate than the ones made by hand, in the offline mode, do to say.
How do scientists traditionally map soil types? They go to a location, and make a cross-section soil sample on a most characteristic landform; the sample looks like a pit but with steps. They dig it until all soil layers (genetic horizons) can be distinguished by color gradients and other visual features under natural light. And once researchers have a set of these soil sections in which soil types have already been studied, they manually build a map based on them. If we gave the same dataset to 10 soil scientists, they would make 10 similar maps that are slightly different in detail.
With the mathematical approach, one can feel the difference. By analyzing soil profile data with an algorithm considering digital elevation model (DEM) derivatives and climatic characteristics, we would get a map of higher accuracy than the one done conventionally. This approach also allows for getting a map of the so-called uncertainties or an error map that indicates probabilities of finding one or another soil at one point.
What Are The Common Use Cases Of Digital Soil Mapping?
The first use case is making soil property maps — maps illustrating quantitative characteristics of soil (e.g., humus, Nitrogen content, pH) and showing spatial distribution of these properties. And these properties can be defined even within one soil type.
The second use case is creating accurate soil type maps. Soil type (or soil class) is an example of a categorical characteristic. It’s described not quantitatively (by a number) but qualitatively (in words: Chernozems, Podzols, Kastanozems). Creating these maps is a standalone approach and entails using specific adaptations of algorithms.
Creating soil type maps refers to the most general level of soil classification. The classification includes the following taxonomic units: type, subtype, genus, lithological series, species, variant, and variety. But we can model soil both at the highest level (soil types) and lower ones, down to the very bottom, getting more detailed maps. Modeling soil cover at different levels refers to mapping taxonomic soil units.
In 2019, we built a map of Ukraine’s agro-production soil groups, and it’s an example of such an approach. Agro-production groups of soils are an artificial classification created for agriculture. It combines levels of taxonomic soil units. Ukrainian soils belong to 222 classes, each with specific agricultural characteristics. We’re very proud of this project as it took researchers 40 years of field research to make a previous (original) version of this map, and the map covered only two-thirds of the country. We completed the work for the entire country in 14 months. The mentioned duration was due to the development of the necessary tools for this kind of modeling, and maps for other countries can already be modeled many times faster.
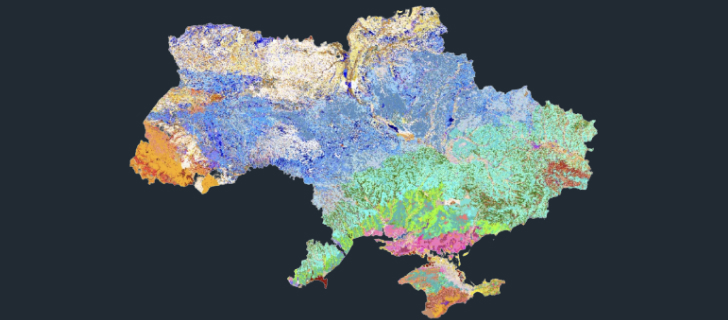
The technology of building soil property maps is a basis for making agrochemical cartograms (they show the amount of nutrient elements for plants) used for crop yield planning and calculation of mineral fertilizer doses for future yields. And a fertilizer application map is based on insights about productivity of field zones (a productivity map) that are extracted via analysis of fields with vegetation indices. That’s how these indicators are interconnected.
You Talked About Soil Layers, Meaning Each Has Its Physical, Chemical, And Biological Properties. Do Digital Soil Maps Depict Only Topsoil?
The maps I talked about before illustrate only topsoil. However, it’s possible to study and describe soil layers placed deeper than 30 cm (30 cm is a topsoil depth) by making volumetric maps. Unlike 2-dimensional maps where every pixel corresponds to a particular land area, these maps are in voxels, the so-called volumetric pixels.
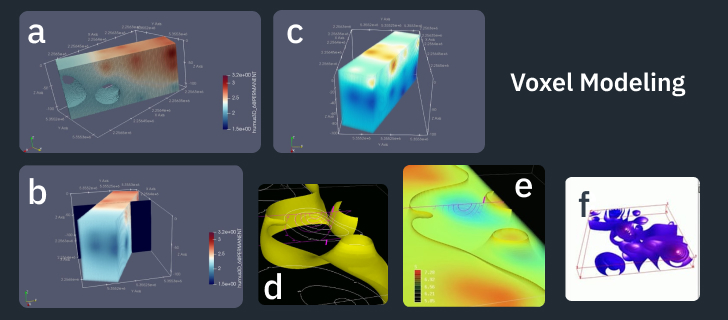
Soil morphometry is a new line of research that focuses on modeling changes in soil properties within soil volume and visualizing these findings in 3D maps.
Plant roots can grow as deep as 1–3 meters (e.g., sunflower, alfalfa, lupine), so the information on the ratio of elements in all soil space lets farmers significantly improve crop nutrition with fertilizers. There is a dedicated softwarе (e.g., SimRoot, SPACSYS, R-SWMS, ROOTMAP, RootTyp) for modeling crops’ root systems on a field scale using voxel data. That way, researchers can formulate recommendations on the time and depth of fertilizer application (it’s possible to apply fertilizer to a depth of 60 cm.)
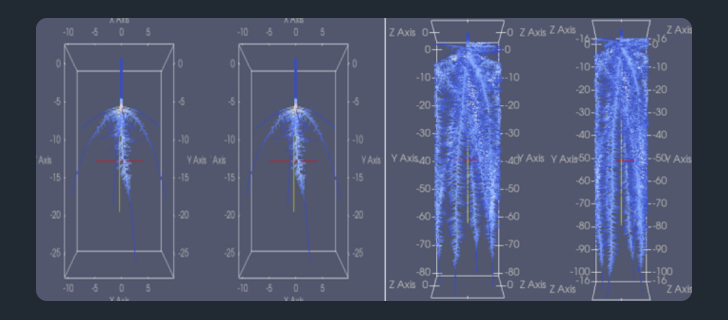
Developing a voxel model of a territory with waterlogged soil and a drainage system can also help farmers optimize its water balance.
Soil Scientists Can Visualize Information On Soils In The Form Of 2D And 3D Maps. What Else For Is Modeling In This Line Of Research Can Be Used?
It can be used for soil degradation calculations. For example, researchers can model water erosion processes to define how much soil will be washed away during a rain on a specific land cover (grass, corn, buckwheat). It will help them estimate risks of soil damage based on what crop will be grown, predict where exactly water erosion can occur and plan how to deal with it if it happens.
This slide with a digital sediment flow map shows places of washed-away particles and locations of their maximum concentration. You can see that the model, rainfall, and rain distribution are almost identical to the photo in the right corner.
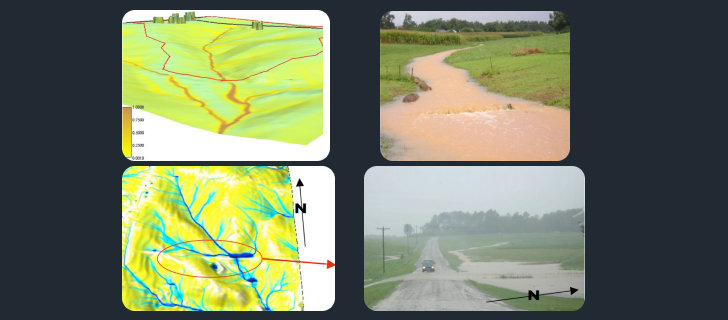
Wind erosion calculation requires more variables to take into account, for example, wind strength and intensity, land cover (seed crop or cover crop), the presence or absence of a windbreak strip, and so on.
Are There Emerging Soil Research Methods Worth Mentioning That You Would Like To Start Using In Your Daily work? And If Yes, What Are The Constraints Of Using Them?
Yes, such methods exist. Besides voxel modeling of soil properties currently used only in scientific publications, I consider the direction that belongs to modeling/mapping of taxonomic soil units, namely disaggregation of conventional soil maps, to be very promising. The soil in large areas of many countries hasn’t been researched (it’s costly), so existing soil maps are based on relatively incomplete information. At the same time, they were built by soil scientists with great practical experience.
Using the power of modern algorithms and geoinformation technologies, specialists can analyze these somewhat schematic maps. And then, relying on additional data (remote sensing data, vegetation indices values, digital elevation model and its derivatives), one can build a highly detailed soil cover model. However, those working in this line of research can struggle to find scanned legacy maps of given countries and regions in sufficient resolution.
I also see excellent prospects in developing technology for predicting and measuring the accumulation of organic carbon in agricultural soils and its dynamics using multispectral optical satellites and bipolar SAR sensors. The accumulation of organic carbon in soils occurs through the capture (sequestration) of carbon dioxide from the atmosphere by plants.
This approach is very valuable not only in terms of benefits for the soil, but also in the fight against global warming. It’s necessary to quantify the relationship between soil measurements and a whole range of additional data to develop such models. I consider the creation of this technology that allows obtaining sufficiently accurate results to be one of the most important and challenging tasks to implement. In addition to algorithmic complexity, a lack of soil data measured in the laboratory makes the problem almost unsolvable.
About the author:
Vasyl Cherlinka is a Doctor of Biosciences specializing in pedology (soil science), with 30 years of experience in the field. He attended the engineering college in Ukraine and received his degree in agrochemistry, agronomy and soil science in the Chernivtsi National University. Since 2018, Dr. Cherlinka has been advising EOSDA on problems in soil science, agronomy, and agrochemistry.
Recent articles
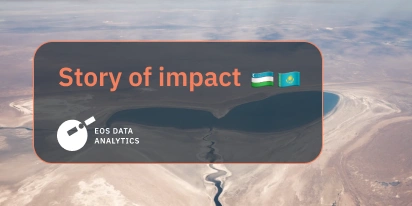
The Aral Sea’s Rebirth: Hope Blooms
This story of impact explores the Aral Sea's restoration, highlighting EOS Data Analytics' satellite insights, and showcasing collaborative efforts to revive a vital ecosystem.
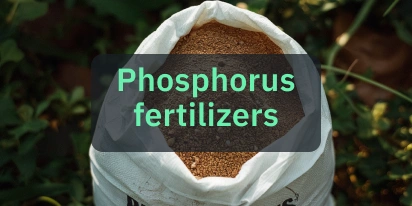
Phosphorus Fertilizers: Types And Role In Agriculture
Key to optimizing crop yields and quality, phosphorus fertilizers are indispensable in agriculture. Explore their types, application methods, and environmental impact in our comprehensive overview.
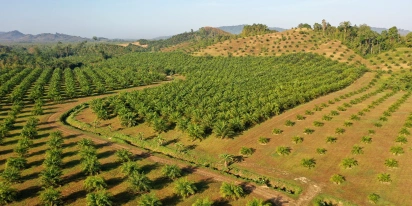
Oil Palm Farming In Southeast Asia: Sustainability Path
Oil palm cultivation in Indonesia, Malaysia, and Thailand forms the foundation of their economies. But plenty of related environmental issues force producers to drastically change their approaches.